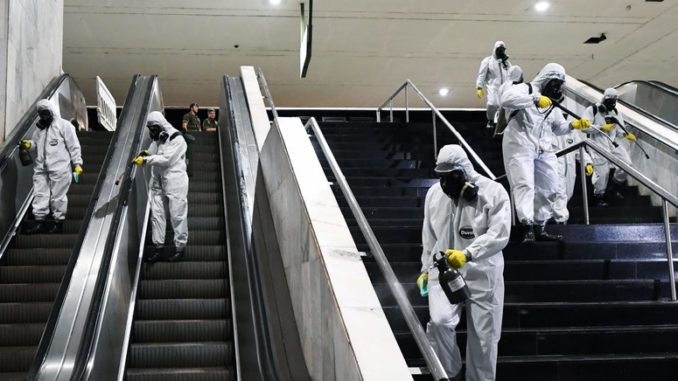
That’s because the innate immune system is also responsible for calling in your next, and more sophisticated line of defense—your adaptive, or acquired, immune system. This is the smart system, the one that can change and adjust, build new defenses to deal with specific threats, and then hold those protections in reserve in case their corresponding threats return. It also regulates the innate immune system. Peptides called cytokines serve as messengers, letting your immune responses know when it’s time to accelerate or pull back.
Late last December, fewer than three months before Neal Browning and the three other first vaccine trial participants offered their arms for injection, Jason McLellan, who runs a molecular biosciences laboratory at the University of Texas at Austin, started hearing about a new respiratory pathogen that had just emerged in Wuhan, China. Given the symptoms, he wondered if it might be a coronavirus.
McLellan had done his postdoc at the Vaccine Research Center, working with Barney Graham. When he finished in 2013, shortly after the emergence of MERS, he talked to Graham about what he should do next. They agreed there was a family of viruses calling out for further study: “We thought it was clear that there would be additional coronavirus outbreaks.”
McLellan started his own lab, which focused on understanding the protein structures of just two families of RNA viruses: Pneumoviridae, such as respiratory syncytial virus, which widely infects infants and children, and Coronaviridae, whose spike-shaped proteins are now infamous. The spike, his team found, operated similarly across all the coronaviruses they studied. The lab members began to create three-dimensional maps of the spikes, so detailed that they showed the location of each atom. (They used a technique called cryo-electron microscopy: essentially using liquid nitrogen to freeze molecules in place, and then using a bombardment of electrons to capture their structure.) They knew that blueprints of the structures that the adaptive immune system would have to learn to neutralize could be invaluable for future efforts to make vaccines.
But there was a complication: The spikes kept transforming. It was their nature. They needed to be one shape to bind to a cell and then another to enter it; once this fusion began, what started out looking like a mushroom changed—losing its cap, elongating, and twisting into something new. It might do little good for the immune system to learn to recognize this post-fusion structure, so McLellan’s lab started researching ways to stabilize the protein, locking it into the shape that it actually used to break into cells. They mapped which parts of the structure changed and which didn’t, and they found that they could use carefully engineered genetic mutations as if they were staples, locking down regions of the spike that wanted to move around by binding them to regions that did not.
In early January, McLellan was snowboarding with his family in Utah when he got a call from Graham. He was calling about the disease circulating in Wuhan: “It looks like this is a coronavirus,” Graham said. “Are you ready to put everything together and race on this?”
“Yes,” McLellan replied. “We’re ready.”
On January 10, one day before China announced its first death from the new disease—at that point it was known to have sickened just 41 people—a consortium of researchers published a draft sequence of the genome of the new virus. Labs across the world got to work. In Texas, it was Friday night, but McLellan and his team didn’t wait. SARS-CoV-2 was a new version of a familiar problem; they could apply the stabilizing mutations they’d been developing right away. McLellan messaged Daniel Wrapp, a grad student, on WhatsApp. The next morning, Wrapp and Kizzmekia Corbett, the scientific lead of the Vaccine Research Center team that studies coronaviruses, got to work using mutations their colleague Nianshuang Wang had already identified. Within an hour or two, they had a genetic sequence for a stabilized version of the new virus’s spike protein.
As their MERS collaboration continued, scientists at the Vaccine Research Center and Moderna had been exploring whether it would be possible, if a viral epidemic were to break out, to work together and use Moderna’s mRNA platform to make a rapid vaccine. Within a day of getting the sequence of the new virus, they decided to try. In those early days, the outbreak was still widely expected to be contained. Rather than a world-changing pathogen, says Moderna’s president, Stephen Hoge, the virus at first seemed like an interesting opportunity to test the potential of their collaboration and their technology.
Even with the head start, beginning the trials so quickly required a sprint. The news about the virus’s spread, and its effects on those it infected, kept getting scarier. It was soon clear that more was riding on the vaccine than anyone had initially realized. Within two weeks, scientists at Moderna, without being asked, were staying late, working weekends. Corbett’s team started growing spike proteins and stocking freezers with vials. They immunized mice with the vaccine, then tested their blood for antibodies. A clinical batch was ready by February 7, tested and shipped by February 24, and green-lit for human testing by March 4. (It was a coincidence that the human trials began in what had, by March, become the first hot spot in the US; Kaiser Permanente Washington Health Research Institute had been selected to conduct them in late January.) There was never a singular moment, says Hoge, when he realized the researchers had begun an 18-month marathon. Instead, “it felt like every day, can you run faster, can you run faster, can you run faster?”
In a few weeks, they’d return for another injection—a booster to juice their immune systems. By then, to calibrate the body’s response, two other cohorts of volunteers would have received their doses: injections of four and 10 times more vaccine that Haller and Browning received. The trial would have expanded to include volunteers considered both “older” and “elderly,” the ones who would need a vaccine the most.
But all that waited somewhere in the deeply uncertain future. For now, almost three weeks after getting his first shot, Browning sat on the deck behind his house, watching a parade of hummingbirds come and go from his feeders, their wings beating so fast he couldn’t see them but still holding them aloft. He thought, yet again, about what his cells might be invisibly up to. There were a lot of possibilities. His B and T cells might be getting more efficient at fighting SARS-CoV-2 all the time; Crotty’s research has shown that, after a month, new generations of immune cells might be 1,000 or even 10,000 times better at binding to a pathogen than they were on the day of the shot. Or, it was possible that even now, all that carefully constructed RNA could be degrading away, leaving behind no real sign that it had ever been introduced.
Browning continued to be an optimist. When he pictured his immune system, he imagined a cadre of his own personal armed guards, sentries out on patrol. Maybe their training against their newest adversary had gone exactly as the whole world hoped. “Maybe,” he says, “my body saw it, and fought it off, and it’s done.”
Let us know what you think about this article. Submit a letter to the editor at [email protected].
Leave a Reply